Chemical - Bonding-6
(b) Bond Length
It has already been discussed that two bonded atoms in a molecule remain held up at a certain distance from each other. They cannot approach too close because it leads to repulsive interactions and potential energy of system increases. This minimum distance between the bonded atoms is referred to as bond length. Thus, bond length may be defined as the average distance between the centres of nuclei of the two bonded atoms in a molecule. Bond length is usually expressed in Angstrom units (Ã…) or picometers (pm) and it can be determined experimentally by X-ray diffraction and and other spectroscopic techniques.
Bond length depends upon the size of the atoms and nature of bonds.
(i) Bond length increases with the increase in the size of the atoms, e.g., bond length between hydrogen and chlorine atoms in HCl molecule is 127 pm whereas bond length between carbon and chlorine atoms is C—Cl bond 177 pm.
(ii) Bond length decreases with the multiplicity of bonds. It is because of the fact that larger the number of electrons shared by the two atoms greater will be attractive force between electrons and the nuclei and consequently, lesser is the bond length. For example, bond length of C—C bond is 154 pm whereas that of C = C bond is 134 pm. Bond lengths of some common bonds are given in Table below.
Table below. Bond Lengths of Some Common Bonds
(c) Bond Angle
We know that covalent bonds are formed by overlapping of atomic orbitals. Due to directional character of atomic orbitals, the covalent bonds in a molecule are oriented in specified directions. The bond angle is defined as the average angle between the lines representing the orbitals containing the bonding electrons.
Bond angle is expressed in degree/minute/seconds. For example, H—C—H bond angle in CH4 molecule is 109° 28¢. Similarly, F—B—F bond angle in BF3 is 120° and H—N—H bond angle in NH3 molecule is 107°.
The bond angles in CH4, NH3, H2O and BF3 molecules are shown below in Figure below.
Concept of Hybridisation
It has already been pointed out that covalency of an element is equal to the number of half-filled orbitals present in the valence shell of its atoms. On applying this concept to carbon, we find that the valency of carbon should be equal to 2 because it has only two half-filled orbitals in the valence shell.
6C : 1s2, 2s2, 2px1, 2py1, 2pz0
In the same way, the valency of beryllium should be zero and that of boron should be one as is evident from their ground state configurations.
4Be : 1s2, 2s2, 2p0
5B : 1s2, 2s2, 2px1, 2py0, 2pz0
Contrary to this, carbon atom always exhibits a valency of four while beryllium and boron exhibit valency of two and three respectively.
In order to explain the observed valencies of Be, B and C, it is assumed that these atoms acquire excited states before participating in bonding. In the excited state the electron pair present in 2s-orbital gets unpaired and one of the electrons is promoted to vacant 2p-orbital. For example, the simple excited state configurations of Be, B and C are shown below :
![]() |
![]() |
![]() |
Thus, concept of excitation or promotion of electrons could very well explain the valency of beryllium, boron and carbon as 2, 3 and 4 respectively.
The energy required for excitation is compensated by the energy released during bond formation.
Let us now study the formation of methane from the excited state configuration of carbon.
Carbon uses its four half-filled orbitals for the axial overlap with 1s orbitals of four different H atoms as shown below:
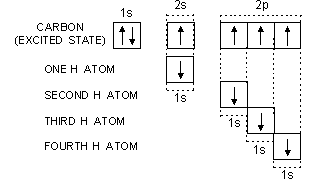
It is quite evident that the four C—H bonds in CH4 (methane) should not be equivalent. Three C—H bonds should be sigma p-s bonds and one C—H bond should be sigma s-s bond. These bonds should be different so far as their (i) strength, (ii) bond length and (iii) bond angle are concerned. The experimental facts about methane reveal that all the C—H bonds in the molecule are equivalent. Their bond length is same i.e., 109 pm and the bond angle H—C—H is 109° 28'. In order to explain these experimental facts and the equivalency of bonds, a concept called hybridisation is introduced.
According to this concept, valence orbitals of the atom intermix to give rise to another set of equivalent orbitals before the formation of bonds. These orbitals are called hybrid orbitals or hybridised orbitals and the phenomenon is referred to as hybridisation. Thus, hybridisation may be defined as the phenomenon of intermixing of atomic orbitals of slightly different energies of the atom (by redistributing their energies) to form new set of orbitals of equivalent energies and identical shape.
In case of carbon atoms, one orbital of 2s-level and three orbitals of 2p-level intermix at the time of participation in bonding to produce four equivalent sp3 hybridised orbitals. These hybrid orbitals overlap axially with 1s orbitals of four H atoms to form four equivalent C—H bonds in methane.
Hybridization
(1) In hybridization, orbitals of almost equal energies mix up to give new orbitals of another space and identical energies. If the energy difference between orbitals is high e.g., 1s and 2p or 2s and 3p, hybridization is not possible. If the energy difference between orbitals is very little e.g., between 2s and 2p or 3s and 3p, hybridization is possible.
(2) The number of orbitals before and after hybridization remains the same.
(3) The type of hybridization is not fixed for an element; rather, it depends on the chemical environment. In different conditions an element may hybridize in different ways. For example, carbon shows sp3, sp2 and sp hybridization in CH4 , C2H4 and C2H2, respectively.
(4) In hybridization, orbitals are used and not electrons, so completely filled, half-filled or empty orbitals can also take part in hybridization. As far as possible, the electrons are shown in the orbitals in such a way that they remains minimum number of paired electrons.
(5) The hybridized orbitals mutually repel each other. The repulsion depends on the number of lone pairs and bond-pairs.
(6) Hybridization is an hypothetical concept which is utilised to explain the experimental observations.
(7) Due to force of repulsion, hybrid orbitals try to remain apart at a maximum possible distance. The order of decreasing force of repulsion is as follows :
lone pair-lone pair > lone pair-bond pair > bond pair-bond pair.
(8) Hybrid orbitals are more directional than the atomic orbitals, hence they form more strong bonds. We know that, greater the overlapping, stronger is the bond formed. This probability is comparatively higher in more directional orbits. The order of bond strength is as under :
sp < sp2 < sp3 < sp3d < sp3d2
(9) The properties of hybrid orbitals taking part in hybridization are in the same ratio in which the orbitals unite. For example, sp hybrid orbitals have 50% properties of s and 50% properties of p-orbital. sp3 hybrid orbitals have 25% properties of s and 75% properties of p-orbital.
(10) Single electron which take part in hybridisation always represent bond.
(11) Electron pair which take part in hybridisation express lone pair of electron.
(12) Single electron which do not take part in hybridisation actually represent bond of system and forms
p—p
, d
— p
or d
—d
type bonding.
(13) The hybrid orbital has electron density concentrated on one side of the nucleus so one lobe is relatively larger than other.
(14) Hybridisation of any species can be find out by the following formula.
Number of hybrid orbital or steric number = number of Bonds + number of lone pair
Types of Hybridization and Shapes of Molecules
There are many different types of hybridisation depending upon the type of orbitals involved in mixing such as sp3, sp2, sp, sp3d, sp3d2, etc.
Let us now discuss various types of hybridisation along with some examples with reference to the compounds of carbon, boron and beryllium.
(i) sp3 hybridisation. The type of hybridisation involves the mixing of one orbital of s-sub-level and three orbitals of p-sub-level of the valence shell to form four sp3 hybrid orbitals of equivalent energies and shape. Each sp3 hybrid orbital has 25% s-character and 75% p-character. These hybridised orbitals tend to lie as far apart in space as possible so that the repulsive interactions between them are minimum. The four sp3 hybrid orbitals are directed towards the four corners of a tetrahedron. The angle between the sp3 hybrid orbitals is 109.5° (Figure below).
sp3 hybridisation is also known as tetrahedral hybridisation. The molecules in which central atom is sp3 hybridised and is linked to four other atoms directly, have tetrahedral shape. Let us study some examples of molecules where the atoms assume sp3 hybrid state.
1. Formation of methane (CH4). In methane carbon atom acquires sp3 hybrid states as described below :
Here, one orbital of 2s-sub-shell and three orbitals of 2p-sub-shell of excited carbon atom undergo hybridisation to form four sp3 hybrid orbitals. The process involving promotion of 2s-electron followed by hybridisation is shown in figure below.
As pointed out earlier the sp3 hybrid orbitals of carbon atom are directed towards the corners of regular tetrahedron. Each of the sp3 hybrid orbitals overlaps axially with half-filled 1s-orbital of hydrogen atom constituting a sigma bond figure below.
Because of sp3hybridisation of carbon atom, CH4 molecule has tetrahedral shape.
2. Formation of ethane (CH3—CH3). In ethane both the carbon atoms assume sp3 hybrid state as shown in figure below. One of the hybrid orbitals of carbon atom overlaps axially with similar orbitals of the other carbon atom to form sp3-sp3 sigma bond. The other three hybrid orbitals of each carbon atom are used in forming sp3-s sigma bonds with hydrogen atoms as described below :
Each C—H bond in ethane is sp3-s sigma bond with bond length 109 pm. The C—C bond is sp3-sp3 sigma bond with bond length 154 pm.
(ii) sp2 hybridisation. This type of hybridisation involves the mixing of one orbital of s-sub-level and two orbitals of p-sub-level of the valence shell to form three sp2 hybrid orbitals. These sp2 hybrid orbitals lie in a plane and are directed towards the corners of equilateral triangle (Figure below). Each sp2 hybrid orbital has one-third s-character and two-third p-character. sp2 hybridisation is also called trigonal hybridisation. The molecules in which central is sp2 hybridised and is linked to three other atoms directly have triangular planar shape.
Let us study some examples of the molecules which involve sp2 hybridisation.